PS1: Physical Modelling - Fluid Properties and Compositional Modelling
Monday, September 5, 2022 |
5:40 PM - 6:50 PM |
Foyer & Room 1.4 |
Speaker
Sofiane Achour
The University of Texas at Austin
A New Model of Compositional Transport in Sorbed Layers for Multicomponent Multiphase Simulation of Tight Reservoirs
5:40 PM - 5:45 PMSummary
The main challenge in modeling compositional transport in tight formations is to include the complex interaction of chemical species with rock surfaces. Such interactions result in compositional variations near the surfaces, which can significantly affect the compositional diffusive transport through tight pores. This paper presents the implementation of a new framework for modeling multicomponent diffusive transport in the central and near-wall sorbed layers of pores. The importance of the inclusion of sorption and capillary pressure is demonstrated in case studies.
The new method in this paper iteratively computes the fluid compositions in the central and sorbed layers subject to a wall chemical potential by a rapid approximation of the Multicomponent Potential Theory of Adsorption (MPTA). This calculation uses a nested loop of flash calculations to minimize the Helmholtz free energy including the effect of capillary pressure for each fluid layer in a pore. The in-house simulator computes the mass transfer driven by fugacity gradient based on the dusty gas model for the central and near-wall layers of pores. This simulator uses a partially implicit formulation to solve the multiphase multicomponent mass transfer equations including the sorbed layer, where only the driving forces are computed implicitly.
The two-region approximation of MPTA is validated against the MPTA calculation using 100 regions discretizing the pore volume between the pore center and the pore wall. The multicomponent Langmuir model is the most often used for computing the sorbed excess in micropores. However, results show that the Langmuir model becomes physically inconsistent at high pressures. The new approach developed in this research is sufficiently flexible and reasonably accurate for modeling the multicomponent sorption at high pressures.
The diffusion simulation with the rapid MPTA showed that the sorption and capillary pressure caused the compositional segregation between the central and sorbed layers. The segregation enhanced the rates of methane injection and n-decane production in their counter-current diffusion. Methane (the lightest) is transported deep into the reservoir by diffusing through the central layer while n-decane (the heaviest) is diffused primarily through the sorbed layer. n-Butane (the intermediate) did not show preferential partitioning into either layer, resulting in relatively inefficient transport. In the absence of sorption and capillary pressure, the countercurrent diffusion occurred between methane (the lightest) and n-butane (the intermediate) while n-decane (the heaviest) remained nearly immobile. That is, whether the simulation considers the surface-fluid interactions, such as sorption and capillary pressure, can substantially affect the compositional transport (e.g., produced fluid composition) through tight porous media.
The new method in this paper iteratively computes the fluid compositions in the central and sorbed layers subject to a wall chemical potential by a rapid approximation of the Multicomponent Potential Theory of Adsorption (MPTA). This calculation uses a nested loop of flash calculations to minimize the Helmholtz free energy including the effect of capillary pressure for each fluid layer in a pore. The in-house simulator computes the mass transfer driven by fugacity gradient based on the dusty gas model for the central and near-wall layers of pores. This simulator uses a partially implicit formulation to solve the multiphase multicomponent mass transfer equations including the sorbed layer, where only the driving forces are computed implicitly.
The two-region approximation of MPTA is validated against the MPTA calculation using 100 regions discretizing the pore volume between the pore center and the pore wall. The multicomponent Langmuir model is the most often used for computing the sorbed excess in micropores. However, results show that the Langmuir model becomes physically inconsistent at high pressures. The new approach developed in this research is sufficiently flexible and reasonably accurate for modeling the multicomponent sorption at high pressures.
The diffusion simulation with the rapid MPTA showed that the sorption and capillary pressure caused the compositional segregation between the central and sorbed layers. The segregation enhanced the rates of methane injection and n-decane production in their counter-current diffusion. Methane (the lightest) is transported deep into the reservoir by diffusing through the central layer while n-decane (the heaviest) is diffused primarily through the sorbed layer. n-Butane (the intermediate) did not show preferential partitioning into either layer, resulting in relatively inefficient transport. In the absence of sorption and capillary pressure, the countercurrent diffusion occurred between methane (the lightest) and n-butane (the intermediate) while n-decane (the heaviest) remained nearly immobile. That is, whether the simulation considers the surface-fluid interactions, such as sorption and capillary pressure, can substantially affect the compositional transport (e.g., produced fluid composition) through tight porous media.
Ms Fangning Zheng
University of Southern California
Optimization of CO2 Storage and Leakage through Caprock Fracturing using Coupled Flow-Geomechanics-Fracturing Simulation
5:45 PM - 5:50 PMSummary
Geologic CO2 storage (GCS) has been recognized as one of the major mitigation strategies for reducing atmospheric CO2 emission. During CO2 injection into the storage reservoirs, pressure increase induces stress changes in and around the injection reservoir, which may cause various geomechanical hazards such as caprock failure, ground surface uplifting, and induced seismicity. Caprock failure could result in creating a leakage pathway for CO2 to leak from the storage reservoir to shallower aquifers and even to the atmosphere. Prior studies related to CO2 storage and leakage have focused exclusively on the issues of modeling these processes. However, there is little investigation into how caprock fracturing and associated leakage may affect the storage performance at a site. As a result, there is limited knowledge on how to control injection wells to optimize storage in presence of such risks.
We focus on the CO2 storage optimization and leakage mitigation problem due to injection-induced changes in pressure and stress. The objective is to minimize the CO2 leakage through caprock fracturing given a fixed amount of total CO2 injection. To this end, we develop a workflow for optimizing CO2 storage under the leakage risk by incorporating coupled flow-geomechanics-fracturing simulation into a gradient-based optimization algorithm. We build a heterogeneous 3D coupled multiphase flow and geomechanics model for CO2 injection using the CMG GEM package, where the Barton-Bandis Model is used to model the fracturing of caprock during injection period. We quantify the CO2 leakage metric as the total amount of CO2 leaking out of the injection reservoir into shallower aquifers through fractures in the caprock caused by pore pressure increase. An optimization formulation is established to minimize the total CO2 leakage amount by finding optimal well control schedules with a fixed amount of total CO2 injection.
We show that flow-only simulation has limited application to the caprock fracturing problem. For a fixed total CO2 injection into an aquifer with heterogenous flow properties, the proposed optimization algorithm finds the injection schedule that distributes the pressure in the reservoir to minimize the risk of CO2 leakage. While a flow-only modeling approach cannot be used for injection optimization under leakage risk, the coupled modeling approach can model the fracturing process and estimate the corresponding leakage rates. The use of coupled flow-geomechanics simulation enables CO2 leakage modeling and provides a tool for optimization of storage efficiency while minimizing the risk of caprock integrity failure and CO2 leakage.
We focus on the CO2 storage optimization and leakage mitigation problem due to injection-induced changes in pressure and stress. The objective is to minimize the CO2 leakage through caprock fracturing given a fixed amount of total CO2 injection. To this end, we develop a workflow for optimizing CO2 storage under the leakage risk by incorporating coupled flow-geomechanics-fracturing simulation into a gradient-based optimization algorithm. We build a heterogeneous 3D coupled multiphase flow and geomechanics model for CO2 injection using the CMG GEM package, where the Barton-Bandis Model is used to model the fracturing of caprock during injection period. We quantify the CO2 leakage metric as the total amount of CO2 leaking out of the injection reservoir into shallower aquifers through fractures in the caprock caused by pore pressure increase. An optimization formulation is established to minimize the total CO2 leakage amount by finding optimal well control schedules with a fixed amount of total CO2 injection.
We show that flow-only simulation has limited application to the caprock fracturing problem. For a fixed total CO2 injection into an aquifer with heterogenous flow properties, the proposed optimization algorithm finds the injection schedule that distributes the pressure in the reservoir to minimize the risk of CO2 leakage. While a flow-only modeling approach cannot be used for injection optimization under leakage risk, the coupled modeling approach can model the fracturing process and estimate the corresponding leakage rates. The use of coupled flow-geomechanics simulation enables CO2 leakage modeling and provides a tool for optimization of storage efficiency while minimizing the risk of caprock integrity failure and CO2 leakage.
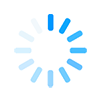